Miocene
Miocene | |||||||||||
---|---|---|---|---|---|---|---|---|---|---|---|
![]() Map of Earth as it appeared 20 million years ago during the Miocene, Burdigalian Age[citation needed] | |||||||||||
Chronology | |||||||||||
| |||||||||||
Etymology | |||||||||||
Name formality | Formal | ||||||||||
Usage information | |||||||||||
Celestial body | Earth | ||||||||||
Regional usage | Global (ICS) | ||||||||||
Time scale(s) used | ICS Time Scale | ||||||||||
Definition | |||||||||||
Chronological unit | Epoch | ||||||||||
Stratigraphic unit | Series | ||||||||||
Time span formality | Formal | ||||||||||
Lower boundary definition |
| ||||||||||
Lower boundary GSSP | Lemme-Carrosio Section, Carrosio, Italy 44°39′32″N 8°50′11″E / 44.6589°N 8.8364°E | ||||||||||
Lower GSSP ratified | 1996[4] | ||||||||||
Upper boundary definition | Base of the Thvera magnetic event (C3n.4n), which is only 96 ka (5 precession cycles) younger than the GSSP | ||||||||||
Upper boundary GSSP | Heraclea Minoa section, Heraclea Minoa, Cattolica Eraclea, Sicily, Italy 37°23′30″N 13°16′50″E / 37.3917°N 13.2806°E | ||||||||||
Upper GSSP ratified | 2000[5] |
The Miocene (/ˈmaɪ.əsiːn, -oʊ-/ MY-ə-seen, -oh-)[6][7] is the first geological epoch of the Neogene Period and extends from about 23.03 to 5.333 million years ago (Ma). The Miocene was named by Scottish geologist Charles Lyell; the name comes from the Greek words μείων (meíōn, "less") and καινός (kainós, "new")[8][9] and means "less recent" because it has 18% fewer modern marine invertebrates than the Pliocene has.[10] The Miocene followed the Oligocene and preceded the Pliocene.
As Earth went from the Oligocene through the Miocene and into the Pliocene, the climate slowly cooled towards a series of ice ages.[11][12] The Miocene boundaries are not marked by distinct global events but by regionally defined transitions from the warmer Oligocene to the cooler Pliocene Epoch.
During the Early Miocene, Afro-Arabia collided with Eurasia, severing the connection between the Mediterranean and Indian Oceans, and allowing the interchange of fauna between Eurasia and Africa, including the dispersal of proboscideans and hominoids[13] into Eurasia. During the late Miocene, the connections between the Atlantic and Mediterranean closed, causing the Mediterranean Sea to almost completely evaporate. This event is referred to as the “Messinian salinity crisis”. Then, at the Miocene–Pliocene boundary, the Strait of Gibraltar opened, and the Mediterranean refilled. That event is referred to as the “Zanclean flood”.
Also during the early Miocene (specifically the Aquitanian and Burdigalian Stages), the apes first evolved, began diversifying, and became widespread throughout the Old World. Around the end of this epoch, the ancestors of humans had split away from the ancestors of the chimpanzees and had begun following their own evolutionary path during the final Messinian Stage (7.5–5.3 Ma) of the Miocene. As in the Oligocene before it, grasslands continued to expand, and forests to dwindle. In the seas of the Miocene, kelp forests made their first appearance and soon became one of Earth's most productive ecosystems.[14]
The plants and animals of the Miocene were recognizably modern. Mammals and birds were well established. Whales, pinnipeds, and kelp spread.
The Miocene is of particular interest to geologists and palaeoclimatologists because major phases of the geology of the Himalaya occurred during that epoch, affecting monsoonal patterns in Asia, which were interlinked with glacial periods in the northern hemisphere.[15]
Subdivisions
[edit]
The Miocene faunal stages from youngest to oldest are typically named according to the International Commission on Stratigraphy:[16]
Sub-epoch | Faunal stage | Time range |
---|---|---|
Late Miocene | Messinian | 7.246–5.333 Ma |
Tortonian | 11.63–7.246 Ma | |
Middle Miocene | Serravallian | 13.82–11.63 Ma |
Langhian | 15.97–13.82 Ma | |
Early Miocene | Burdigalian | 20.44–15.97 Ma |
Aquitanian | 23.03–20.44 Ma |
Regionally, other systems are used, based on characteristic land mammals; some of them overlap with the preceding Oligocene and following Pliocene Epochs:
European | North American | South American |
---|---|---|
|
|
|
Paleogeography
[edit]
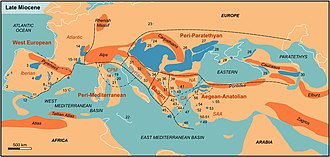
Continents continued to drift toward their present positions. Of the modern geologic features, only the land bridge between South America and North America was absent,[17] although South America was approaching the western subduction zone in the Pacific Ocean, causing both the rise of the Andes and a southward extension of the Meso-American peninsula.[18]
Mountain building took place in western North America, Europe, and East Asia.[19] Both continental and marine Miocene deposits are common worldwide with marine outcrops common near modern shorelines. Well studied continental exposures occur in the North American Great Plains and in Argentina.
The global trend was towards increasing aridity caused primarily by global cooling reducing the ability of the atmosphere to absorb moisture,[20] particularly after 7 to 8 million years ago.[21] Uplift of East Africa in the late Miocene was partly responsible for the shrinking of tropical rain forests in that region,[22] and Australia got drier as it entered a zone of low rainfall in the Late Miocene.[23]
Eurasia
[edit]The Indian Plate continued to collide with the Eurasian Plate, creating new mountain ranges and uplifting the Tibetan Plateau, resulting in the rain shadowing and aridification of the Asian interior.[21] The Tian Shan experienced significant uplift in the Late Miocene, blocking westerlies from coming into the Tarim Basin and drying it as a result.[24]
At the beginning of the Miocene, the northern margin of the Arabian plate, then part of the African landmass, collided with Eurasia; as a result, the Tethys seaway continued to shrink and then disappeared as Africa collided with Eurasia in the Turkish–Arabian region.[19] The first step of this closure occurred 20 Ma, reducing water mass exchange by 90%, while the second step occurred around 13.8 Ma, coincident with a major expansion of Antarctic glaciers.[25] This severed the connection between the Indian Ocean and the Mediterranean Sea and formed the present land connection between Afro-Arabia and Eurasia.[26] The subsequent uplift of mountains in the western Mediterranean region and a global fall in sea levels combined to cause a temporary drying up of the Mediterranean Sea (known as the Messinian salinity crisis) near the end of the Miocene.[27] The Paratethys underwent a significant transgression during the early Middle Miocene.[28] Around 13.8 Ma, during a global sea level drop, the Eastern Paratethys was cut off from the global ocean by the closure of the Bârlad Strait, effectively turning it into a saltwater lake. From 13.8 to 13.36 Ma, an evaporite period similar to the later Messinian salinity crisis in the Mediterranean ensued in the Central Paratethys, cut off from sources of freshwater input by its separation from the Eastern Paratethys. From 13.36 to 12.65 Ma, the Central Paratethys was characterised by open marine conditions, before the reopening of the Bârlad Strait resulted in a shift to brackish-marine conditions in the Central Paratethys, causing the Badenian-Sarmatian Extinction Event. As a result of the Bârlad Strait's reopening, the lake levels of the Eastern Paratethys dropped as it once again became a sea.[29]
The Fram Strait opened during the Miocene and acted as the only throughflow for Atlantic Water into the Arctic Ocean until the Quaternary period. Due to regional uplift of the continental shelf, this water could not move through the Barents Seaway in the Miocene.[30]
The modern day Mekong Delta took shape after 8 Ma.[31] Geochemistry of the Qiongdongnan Basin in the northern South China Sea indicates the Pearl River was a major source of sediment flux into the sea during the Early Miocene and was a major fluvial system as in the present.[32]
South America
[edit]During the Oligocene and Early Miocene, the coast of northern Brazil,[33] Colombia, south-central Peru, central Chile and large swathes of inland Patagonia were subject to a marine transgression.[34] The transgressions in the west coast of South America are thought to be caused by a regional phenomenon while the steadily rising central segment of the Andes represents an exception.[34] While there are numerous registers of Oligocene–Miocene transgressions around the world it is doubtful that these correlate.[33]
It is thought that the Oligocene–Miocene transgression in Patagonia could have temporarily linked the Pacific and Atlantic Oceans, as inferred from the findings of marine invertebrate fossils of both Atlantic and Pacific affinity in La Cascada Formation.[35][36] Connection would have occurred through narrow epicontinental seaways that formed channels in a dissected topography.[35][37]
The Antarctic Plate started to subduct beneath South America 14 million years ago in the Miocene, forming the Chile Triple Junction. At first the Antarctic Plate subducted only in the southernmost tip of Patagonia, meaning that the Chile Triple Junction lay near the Strait of Magellan. As the southern part of Nazca Plate and the Chile Rise became consumed by subduction the more northerly regions of the Antarctic Plate begun to subduct beneath Patagonia so that the Chile Triple Junction advanced to the north over time.[38] The asthenospheric window associated to the triple junction disturbed previous patterns of mantle convection beneath Patagonia inducing an uplift of ca. 1 km that reversed the Oligocene–Miocene transgression.[37][39]
As the southern Andes rose in the Middle Miocene (14–12 million years ago) the resulting rain shadow originated the Patagonian Desert to the east.[40]
Australia
[edit]Far northern Australia was monsoonal during the Miocene. Although northern Australia is often believed to have been much wetter during the Miocene, this interpretation may be an artefact of preservation bias of riparian and lacustrine plants;[41] this finding has itself been challenged by other papers.[42] Western Australia, like today, was arid, particularly so during the Middle Miocene.[43]
Climate
[edit]
Climates remained moderately warm, although the slow global cooling that eventually led to the Pleistocene glaciations continued. Although a long-term cooling trend was well underway, there is evidence of a warm period during the Miocene when the global climate rivalled that of the Oligocene.[citation needed] The climate of the Miocene has been suggested as a good analogue for future warmer climates caused by anthropogenic global warming,[11] with this being especially true of the global climate during the Middle Miocene Climatic Optimum (MMCO),[12][44][45] because the last time carbon dioxide levels were comparable to projected future atmospheric carbon dioxide levels resulting from anthropogenic climate change was during the MMCO.[46]
The Miocene began with the Early Miocene Cool Event (Mi-1) around 23 million years ago, which marked the start of the Early Miocene Cool Interval (EMCI).[47] This cool event occurred immediately after the Oligocene-Miocene Transition (OMT) during a major expansion of Antarctica's ice sheets,[48] but was not associated with a significant drop in atmospheric carbon dioxide levels.[49] Both continental and oceanic thermal gradients in mid-latitudes during the Early Miocene were very similar to those in the present.[50] Global cooling caused the East Asian Summer Monsoon (EASM) to begin to take on its modern form during the Early Miocene.[51] From 22.1 to 19.7 Ma, the Xining Basin experienced relative warmth and humidity amidst a broader aridification trend.[52]
The EMCI ended 18 million years ago, giving way to the Middle Miocene Warm Interval (MMWI), the warmest part of which was the MMCO that began 16 million years ago.[47] As the world transitioned into the MMCO, carbon dioxide concentrations varied between 300 and 500 ppm.[53] Global annual mean surface temperature during the MMCO was about 18.4 °C.[54] MMCO warmth was driven by the activity of the Columbia River Basalts[55][56][57] and enhanced by decreased albedo from the reduction of deserts and expansion of forests.[58] Climate modelling suggests additional, currently unknown, factors also worked to create the warm conditions of the MMCO.[59] The MMCO saw the expansion of the tropical climatic zone to much larger than its current size.[60] The July ITCZ, the zone of maximal monsoonal rainfall, moved to the north, increasing precipitation over southern China whilst simultaneously decreasing it over Indochina during the EASM.[61] Western Australia was at this time characterised by exceptional aridity.[43] In Antarctica, average summer temperatures on land reached 10 °C.[62] In the oceans, the lysocline shoaled by approximately half of a kilometre during warm phases that corresponded to orbital eccentricity maxima.[63] The MMCO ended around 14 million years ago,[47] when global temperatures fell in the Middle Miocene Climate Transition (MMCT).[64] Abrupt increases in opal deposition indicate this cooling was driven by enhanced drawdown of carbon dioxide via silicate weathering.[65] The MMCT caused a sea surface temperature (SST) drop of approximately 6 °C in the North Atlantic.[66] The drop in benthic foraminiferal δ18O values was most noticeable in the waters around Antarctica, suggesting cooling was most intense there.[67] Around this time the Mi3b glacial event (a massive expansion of Antarctic glaciers) occurred.[68] The East Antarctic Ice Sheet (EAIS) markedly stabilised following the MMCT.[69] The intensification of glaciation caused a decoherence of sediment deposition from the 405 kyr eccentricity cycle.[70]

The MMWI ended about 11 Ma, when the Late Miocene Cool Interval (LMCI) started.[47] A major but transient warming occurred around 10.8-10.7 Ma.[71] During the Late Miocene, the Earth's climate began to display a high degree of similarity to that of the present day[according to whom?][citation needed]. The 173 kyr obliquity modulation cycle governed by Earth's interactions with Saturn became detectable in the Late Miocene.[72] By 12 Ma, Oregon was a savanna akin to that of the western margins of the Sierra Nevada of northern California.[73] Central Australia became progressively drier,[74] although southwestern Australia experienced significant wettening from around 12 to 8 Ma.[43] The South Asian Winter Monsoon (SAWM) underwent strengthening ~9.2–8.5 Ma.[75] From 7.9 to 5.8 Ma, the East Asian Winter Monsoon (EAWM) became stronger synchronously with a southward shift of the subarctic front.[76] Greenland may have begun to have large glaciers as early as 8 to 7 Ma,[77][78] although the climate for the most part remained warm enough to support forests there well into the Pliocene.[79] Zhejiang, China was noticeably more humid than today.[80] In the Great Rift Valley of Kenya, there was a gradual and progressive trend of increasing aridification, though it was not unidirectional, and wet humid episodes continued to occur.[81] Between 7 and 5.3 Ma, temperatures dropped sharply again in the Late Miocene Cooling (LMC),[47] most likely as a result of a decline in atmospheric carbon dioxide[82][83][84] and a drop in the amplitude of Earth's obliquity,[85] and the Antarctic ice sheet was approaching its present-day size and thickness. Ocean temperatures plummeted to near-modern values during the LMC;[86] extratropical sea surface temperatures dropped substantially by approximately 7-9 °C.[87] 41 kyr obliquity cycles became the dominant orbital climatic control 7.7 Ma and this dominance strengthened 6.4 Ma.[88] Benthic δ18O values show significant glaciation occurred from 6.26 to 5.50 Ma, during which glacial-interglacial cycles were governed by the 41 kyr obliquity cycle.[89] A major reorganisation of the carbon cycle occurred approximately 6 Ma, causing continental carbon reservoirs to no longer expand during cold spells, as they had done during cold periods in the Oligocene and most of the Miocene.[90] At the end of the Miocene, global temperatures rose again as the amplitude of Earth's obliquity increased,[85] which caused increased aridity in Central Asia.[91] Around 5.5 Ma, the EAWM underwent a period of rapid intensification.[92]
Life
[edit]Life during the Miocene Epoch was mostly supported by the two newly formed biomes, kelp forests and grasslands[according to whom?][citation needed]. Grasslands allow for more grazers, such as horses, rhinoceroses, and hippos. Ninety-five percent of modern plants existed by the end of this epoch[citation needed]. Modern bony fish genera were established.[93] A modern-style latitudinal biodiversity gradient appeared ~15 Ma.[94]
Flora
[edit]The coevolution of gritty, fibrous, fire-tolerant grasses and long-legged gregarious ungulates with high-crowned teeth, led to a major expansion of grass-grazer ecosystems[citation needed]. Herds of large, swift grazers were hunted by predators across broad sweeps of open grasslands, displacing desert, woodland, and browsers[citation needed].
The higher organic content and water retention of the deeper and richer grassland soils, with long-term burial of carbon in sediments, produced a carbon and water vapor sink. This, combined with higher surface albedo and lower evapotranspiration of grassland, contributed to a cooler, drier climate.[96] C4 grasses, which are able to assimilate carbon dioxide and water more efficiently than C3 grasses, expanded to become ecologically significant near the end of the Miocene between 6 and 7 million years ago.[97] The expansion of grasslands and radiations among terrestrial herbivores correlates to fluctuations in CO2.[98] One study, however, has attributed the expansion of grasslands not to a CO2 drop but to the increasing seasonality and aridity, coupled with a monsoon climate, which made wildfires highly prevalent compared to before.[99] The Late Miocene expansion of grasslands had cascading effects on the global carbon cycle, evidenced by the imprint it left in carbon isotope records.[100]
Cycads between 11.5 and 5 million years ago began to rediversify after previous declines in variety due to climatic changes, and thus modern cycads are not a good model for a "living fossil".[101] Eucalyptus fossil leaves occur in the Miocene of New Zealand, where the genus is not native today, but have been introduced from Australia.[102]
Fauna
[edit]−10 — – −9 — – −8 — – −7 — – −6 — – −5 — – −4 — – −3 — – −2 — – −1 — – 0 — |
| |||||||||||||||||||||||||||||


Both marine and continental fauna were fairly modern, although marine mammals were less numerous. Only in isolated South America and Australia did widely divergent fauna exist.
In the Early Miocene, several Oligocene groups were still diverse, including nimravids, entelodonts, and three-toed equids. As in the previous Oligocene Epoch, oreodonts were still diverse, only to disappear in the earliest Pliocene. During the later Miocene mammals were more modern, with easily recognizable canids, bears, red pandas, procyonids, equids, beavers, deer, camelids, and whales, along with now-extinct groups like borophagine canids, certain gomphotheres, three-toed horses, and hornless rhinos like Teleoceras and Aphelos. The late Miocene also marks the extinction of the last-surviving members of the hyaenodonts. Islands began to form between South and North America in the Late Miocene, allowing ground sloths like Thinobadistes to island-hop to North America. The expansion of silica-rich C4 grasses led to worldwide extinctions of herbivorous species without high-crowned teeth.[103] Mustelids diversified into their largest forms as terrestrial predators like Ekorus, Eomellivora, and Megalictis and bunodont otters like Enhydriodon and Sivaonyx appeared. Eulipotyphlans were widespread in Europe, being less diverse in Southern Europe than farther north due to the aridity of the former.[104]
Unequivocally-recognizable dabbling ducks, plovers, typical owls, cockatoos and crows appear during the Miocene. By the epoch's end, all or almost all modern bird groups are believed to have been present; the few post-Miocene bird fossils which cannot be placed in the evolutionary tree with full confidence are simply too badly preserved, rather than too equivocal in character. Marine birds reached their highest diversity ever in the course of this epoch[citation needed].
The youngest representatives of Choristodera, an extinct order of aquatic reptiles that first appeared in the Middle Jurassic, are known from the Miocene of Europe, belonging to the genus Lazarussuchus, which had been the only known surviving genus of the group since the beginning of the Eocene.[105]
The last known representatives of the archaic primitive mammal order Meridiolestida, which dominated South America during the Late Cretaceous, are known from the Miocene of Patagonia, represented by the mole-like Necrolestes.[106][107]
The youngest known representatives of metatherians (the broader grouping to which marsupials belong) in Europe, Asia and Africa are known from the Miocene, including the European herpetotheriid Amphiperatherium, the peradectids Siamoperadectes and Sinoperadectes from Asia,[108][109] and the possible herpetotheriid Morotodon from the late Early Miocene of Uganda.[110]
Approximately 100 species of apes lived during this time[citation needed], ranging throughout Africa, Asia and Europe and varying widely in size, diet, and anatomy. Due to scanty fossil evidence it is unclear which ape or apes contributed to the modern hominid clade, but molecular evidence indicates this ape lived between 18 and 13 million years ago.[111] The first hominins (bipedal apes of the human lineage) appeared in Africa at the very end of the Miocene, including Sahelanthropus, Orrorin, and an early form of Ardipithecus (A. kadabba). The chimpanzee–human divergence is thought to have occurred at this time.[112] The evolution of bipedalism in apes at the end of the Miocene instigated an increased rate of faunal turnover in Africa.[113] In contrast, European apes met their end at the end of the Miocene due to increased habitat uniformity.[114]
The expansion of grasslands in North America also led to an explosive radiation among snakes.[115] Previously, snakes were a minor component of the North American fauna, but during the Miocene, the number of species and their prevalence increased dramatically with the first appearances of vipers and elapids in North America and the significant diversification of Colubridae (including the origin of many modern genera such as Nerodia, Lampropeltis, Pituophis and Pantherophis).[115]
Arthropods were abundant, including in areas such as Tibet where they have traditionally been thought to be undiverse.[116] Neoisopterans diversified and expanded into areas they previously were absent from, such as Madagascar and Australia.[117]


Oceanic
[edit]In the oceans, brown algae, called kelp, proliferated, supporting new species of sea life, including otters, fish and various invertebrates.
Corals suffered a significant local decline along the northeastern coast of Australia during the Tortonian, most likely due to warming seawater.[118]
Cetaceans attained their greatest diversity during the Miocene,[119] with over 20 recognized genera of baleen whales in comparison to only six living genera.[120] This diversification correlates with emergence of gigantic macro-predators such as megatoothed sharks and raptorial sperm whales.[121] Prominent examples are O. megalodon and L. melvillei.[121] Other notable large sharks were O. chubutensis, Isurus hastalis, and Hemipristis serra.
Crocodilians also showed signs of diversification during the Miocene. The largest form among them was a gigantic caiman Purussaurus which inhabited South America.[122] Another gigantic form was a false gharial Rhamphosuchus, which inhabited modern age India. A strange form, Mourasuchus also thrived alongside Purussaurus. This species developed a specialized filter-feeding mechanism, and it likely preyed upon small fauna despite its gigantic size.[123]
The youngest members of Sebecidae, a clade of large terrestrial predatory crocodyliformes distantly related to modern crocodilians, from which they likely diverged over 180 million years ago, are known from the Miocene of South America.[123][124]
The last Desmostylians thrived during this period before becoming the only extinct marine mammal order.
The pinnipeds, which appeared near the end of the Oligocene, became more aquatic. A prominent genus was Allodesmus.[125] A ferocious walrus, Pelagiarctos may have preyed upon other species of pinnipeds including Allodesmus.
Furthermore, South American waters witnessed the arrival of Megapiranha paranensis, which were considerably larger than modern age piranhas.
New Zealand's Miocene fossil record is particularly rich. Marine deposits showcase a variety of cetaceans and penguins, illustrating the evolution of both groups into modern representatives. The early Miocene Saint Bathans Fauna is the only Cenozoic terrestrial fossil record of the landmass, showcasing a wide variety of not only bird species, including early representatives of clades such as moa, kiwi and adzebills, but also a diverse herpetofauna of sphenodontians, crocodiles and turtles as well as a rich terrestrial mammal fauna composed of various species of bats and the enigmatic Saint Bathans Mammal.
Microbiota
[edit]Microbial life in the igneous crust of the Fennoscandian Shield shifted from being dominated by methanogens to being primarily composed of sulphate-reducing prokaryotes. The change resulted from fracture reactivation during the Pyrenean-Alpine orogeny, enabling sulphate-reducing microbes to permeate into the Fennoscandian Shield via descending surficial waters.[126]
Diatom diversity was inversely correlated with carbon dioxide levels and global temperatures during the Miocene. Most modern lineages of diatoms appeared by the Late Miocene.[127]
Oceans
[edit]
There is evidence from oxygen isotopes at Deep Sea Drilling Program sites that ice began to build up in Antarctica about 36 Ma during the Eocene. Further marked decreases in temperature during the Middle Miocene at 15 Ma probably reflect increased ice growth in Antarctica. It can therefore be assumed that East Antarctica had some glaciers during the early to mid Miocene (23–15 Ma). Oceans cooled partly due to the formation of the Antarctic Circumpolar Current, and about 15 million years ago the ice cap in the southern hemisphere started to grow to its present form. The Greenland ice cap developed later, in the Middle Pliocene time, about 3 million years ago.
Middle Miocene disruption
[edit]The "Middle Miocene disruption" refers to a wave of extinctions of terrestrial and aquatic life forms that occurred following the Miocene Climatic Optimum (18 to 16 Ma), around 14.8 to 14.5 million years ago, during the Langhian Stage of the mid-Miocene. A major and permanent cooling step occurred between 14.8 and 14.1 Ma, associated with increased production of cold Antarctic deep waters and a major expansion of the East Antarctic ice sheet.[128] The closure of the Indonesian Throughflow, which caused an accumulation of warm water in the western Pacific that then spread eastward and reduced upwelling in the eastern Pacific, may also have been responsible.[129] A Middle Miocene δ18O increase, that is, a relative increase in the heavier isotope of oxygen, has been noted in the Pacific, the Southern Ocean and the South Atlantic.[128] Barium and uranium became enriched in seafloor sediments.[130]
Impact event
[edit]A large impact event occurred either during the Miocene (23 Ma – 5.3 Ma) or the Pliocene (5.3 Ma – 2.6 Ma). The event formed the Karakul crater (52 km diameter), in Tajikistan which is estimated to have an age of less than 23 Ma[131] or less than 5 Ma.[132]
See also
[edit]References
[edit]- ^ Krijgsman, W.; Garcés, M.; Langereis, C. G.; Daams, R.; Van Dam, J.; Van Der Meulen, A. J.; Agustí, J.; Cabrera, L. (1996). "A new chronology for the middle to late Miocene continental record in Spain". Earth and Planetary Science Letters. 142 (3–4): 367–380. Bibcode:1996E&PSL.142..367K. doi:10.1016/0012-821X(96)00109-4.
- ^ Retallack, G. J. (1997). "Neogene Expansion of the North American Prairie". PALAIOS. 12 (4): 380–390. doi:10.2307/3515337. JSTOR 3515337. Retrieved 2008-02-11.
- ^ "ICS Timescale Chart" (PDF). www.stratigraphy.org.
- ^ Steininger, Fritz F.; M. P. Aubry; W. A. Berggren; M. Biolzi; A. M. Borsetti; Julie E. Cartlidge; F. Cati; R. Corfield; R. Gelati; S. Iaccarino; C. Napoleone; F. Ottner; F. Rögl; R. Roetzel; S. Spezzaferri; F. Tateo; G. Villa; D. Zevenboom (1997). "The Global Stratotype Section and Point (GSSP) for the base of the Neogene" (PDF). Episodes. 20 (1): 23–28. doi:10.18814/epiiugs/1997/v20i1/005.
- ^ Van Couvering, John; Castradori, Davide; Cita, Maria; Hilgen, Frederik; Rio, Domenico (September 2000). "The base of the Zanclean Stage and of the Pliocene Series" (PDF). Episodes. 23 (3): 179–187. doi:10.18814/epiiugs/2000/v23i3/005.
- ^ "Miocene". Dictionary.com Unabridged (Online). n.d.
- ^ "Miocene". Merriam-Webster.com Dictionary. Merriam-Webster.
- ^ See:
- Letter from William Whewell to Charles Lyell dated 31 January 1831 in: Todhunter, Isaac, ed. (1876). William Whewell, D. D., Master of Trinity College, Cambridge: An account of his writings with selections from his literary and scientific correspondence. Vol. 2. London: Macmillan and Co. p. 111.
- Lyell, Charles (1833). Principles of Geology, …. Vol. 3. London, England: John Murray. p. 54. From p. 54: "The next antecedent tertiary epoch we shall name Miocene, from μειων, minor, and χαινος, recens, a minority only of fossil shells imbedded in the formations of this period, being of recent species."
- ^ Harper, Douglas. "Miocene". Online Etymology Dictionary. Retrieved 2016-01-20.
- ^ Lyell, Charles (1833). Principles of Geology, …. Vol. 3. London, England: John Murray. p. 54.
- ^ a b Gibson, M. E.; McCoy, J.; O'Keefe, J. M. K.; Otaño, N. B. Nuñez; Warny, S.; Pound, M. J. (2022). "Reconstructing Terrestrial Paleoclimates: A Comparison of the Co-Existence Approach, Bayesian and Probability Reconstruction Techniques Using the UK Neogene". Paleoceanography and Paleoclimatology. 37 (2): e2021PA004358. Bibcode:2022PaPa...37.4358G. doi:10.1029/2021PA004358. S2CID 245937316.
- ^ a b Steinthorsdottir, M.; Coxall, H. K.; Boer, A. M. de; Huber, M.; Barbolini, N.; Bradshaw, C. D.; Burls, N. J.; Feakins, S. J.; Gasson, E.; Henderiks, J.; Holbourn, A. E.; Kiel, S.; Kohn, M. J.; Knorr, G.; Kürschner, W. M.; Lear, C. H.; Liebrand, D.; Lunt, D. J.; Mörs, T.; Pearson, P. N.; Pound, M. J.; Stoll, H.; Strömberg, C. a. E. (2021). "The Miocene: The Future of the Past". Paleoceanography and Paleoclimatology. 36 (4): e2020PA004037. Bibcode:2021PaPa...36.4037S. doi:10.1029/2020PA004037. S2CID 234434792.
- ^ Gilbert, Christopher C.; Pugh, Kelsey D.; Fleagle, John G. (2020), Prasad, Guntupalli V.R.; Patnaik, Rajeev (eds.), "Dispersal of Miocene Hominoids (and Pliopithecoids) from Africa to Eurasia in Light of Changing Tectonics and Climate", Biological Consequences of Plate Tectonics, Cham: Springer International Publishing, pp. 393–412, doi:10.1007/978-3-030-49753-8_17, ISBN 978-3-030-49752-1, retrieved 2024-10-04
- ^ "BBC Nature - Miocene epoch videos, news and facts". BBC. Retrieved 2016-11-13.
- ^ Zhisheng, An; Kutzbach, John E.; Prell, Warren L.; Porter, Stephen C. (3 May 2001). "Evolution of Asian monsoons and phased uplift of the Himalaya–Tibetan plateau since Late Miocene times". Nature. 411 (6833): 62–66. Bibcode:2001Natur.411...62Z. doi:10.1038/35075035. PMID 11333976. S2CID 4398615.
- ^ "International Chronostratigraphic Chart" (PDF). International Commission on Stratigraphy. Retrieved 12 November 2021.
- ^ Stange, Madlen; Sánchez-Villagra, Marcelo R; Salzburger, Walter; Matschiner, Michael (1 July 2018). "Bayesian Divergence-Time Estimation with Genome-Wide Single-Nucleotide Polymorphism Data of Sea Catfishes (Ariidae) Supports Miocene Closure of the Panamanian Isthmus". Systematic Biology. 67 (4): 681–699. doi:10.1093/sysbio/syy006. PMC 6005153. PMID 29385552.
- ^ Torsvik, Trond H.; Cocks, L. Robin M. (2017). Earth history and palaeogeography. Cambridge, United Kingdom: Cambridge University Press. p. 264. ISBN 978-1-107-10532-4.
- ^ a b Torsvik & Cocks 2017, p. 261-264.
- ^ Torsvik & Cocks 2017, p. 267.
- ^ a b Jia, Yunxia; Wu, Haibin; Zhu, Shuya; Li, Qin; Zhang, Chunxia; Yu, Yanyan; Sun, Aizhi (1 November 2020). "Cenozoic aridification in Northwest China evidenced by paleovegetation evolution". Palaeogeography, Palaeoclimatology, Palaeoecology. 557: 109907. Bibcode:2020PPP...55709907J. doi:10.1016/j.palaeo.2020.109907. S2CID 224891646. Retrieved 30 November 2022.
- ^ Wichura, Henry; Bousquet, Romain; Oberhänsli, Roland; Strecker, Manfred R.; Trauth, Martin H. (June 2010). "Evidence for middle Miocene uplift of the East African Plateau". Geology. 38 (6): 543–546. Bibcode:2010Geo....38..543W. doi:10.1130/G31022.1.
- ^ Mao, Xuegang; Retallack, Gregory (January 2019). "Late Miocene drying of central Australia". Palaeogeography, Palaeoclimatology, Palaeoecology. 514: 292–304. Bibcode:2019PPP...514..292M. doi:10.1016/j.palaeo.2018.10.008. S2CID 135124769.
- ^ Chang, Jian; Glorie, Stijn; Qiu, Nansheng; Min, Kyoungwon; Xiao, Yao; Xu, Wei (28 December 2020). "Late Miocene (10.0~6.0 Ma) Rapid Exhumation of the Chinese South Tianshan: Implications for the Timing of Aridification in the Tarim Basin". Geophysical Research Letters. 48 (3): 1–11. doi:10.1029/2020GL090623. S2CID 233964312. Retrieved 21 May 2023.
- ^ Bialik, Or M.; Frank, Martin; Betzler, Christian; Zammit, Ray; Waldmann, Nicolas D. (20 June 2019). "Two-step closure of the Miocene Indian Ocean Gateway to the Mediterranean". Scientific Reports. 9 (1): 8842. Bibcode:2019NatSR...9.8842B. doi:10.1038/s41598-019-45308-7. PMC 6586870. PMID 31222018.
- ^ Torfstein, Adi; Steinberg, Josh (14 August 2020). "The Oligo–Miocene closure of the Tethys Ocean and evolution of the proto-Mediterranean Sea". Scientific Reports. 10 (1): 13817. doi:10.1038/s41598-020-70652-4. ISSN 2045-2322. PMC 7427807. PMID 32796882. Retrieved 4 September 2023.
- ^ Torsvik & Cocks 2017, p. 259, 267, 287.
- ^ Hohenegger, Johann; Roegl, Fred; Coric, Stjepan; Pervesler, Peter; Lirer, Fabrizio; Roetzel, Reinhard; Scholger, Robert; Stingl, Karl (January 2009). "The Styrian Basin: A key to the Middle Miocene (Badenian/Langhian) Central Paratethys transgressions". Austrian Journal of Earth Sciences. 102 (1): 102–132. Retrieved 29 January 2023.
- ^ Simon, Dirk; Palcu, Dan; Meijer, Paul; Krijgsman, Wout (7 December 2018). "The sensitivity of middle Miocene paleoenvironments to changing marine gateways in Central Europe". Geology. 47 (1): 35–38. doi:10.1130/G45698.1. S2CID 134633409. Retrieved 7 January 2023.
- ^ Lasabuda, Amando P. E.; Hanssen, Alfred; Laberg, Jan Sverre; Faleide, Jan Inge; Patton, Henry; Abdelmalak, Mansour M.; Rydningen, Tom Arne; Kjølhamar, Bent (29 June 2023). "Paleobathymetric reconstructions of the SW Barents Seaway and their implications for Atlantic–Arctic ocean circulation". Communications Earth & Environment. 4 (1): 231. Bibcode:2023ComEE...4..231L. doi:10.1038/s43247-023-00899-y. ISSN 2662-4435. Retrieved 12 October 2023.
- ^ Liu, Chang; Clift, Peter D.; Murray, Richard W.; Blusztajn, Jerzy; Ireland, Thomas; Wan, Shiming; Ding, Weiwei (20 February 2017). "Geochemical evidence for initiation of the modern Mekong delta in the southwestern South China Sea after 8Ma". Chemical Geology. 451: 38–54. Bibcode:2017ChGeo.451...38L. doi:10.1016/j.chemgeo.2017.01.008. ISSN 0009-2541. Retrieved 30 December 2023 – via Elsevier Science Direct.
- ^ Ma, Ming; Chen, Guojun; Zhang, Gongcheng; Rahman, M. Julleh Jalalur; Ma, Xiaofeng (1 May 2022). "Geochemistry and provenance of Oligocene to middle Miocene sandstones in the Qiongdongnan Basin, northern South China Sea". Marine Geology. 447: 106794. Bibcode:2022MGeol.44706794M. doi:10.1016/j.margeo.2022.106794. ISSN 0025-3227. S2CID 247970013. Retrieved 19 September 2023.
- ^ a b Rossetti, Dilce F.; Bezerra, Francisco H.R.; Dominguez, José M.L. (2013). "Late Oligocene–Miocene transgressions along the equatorial and eastern margins of Brazil". Earth-Science Reviews. 123: 87–112. Bibcode:2013ESRv..123...87R. doi:10.1016/j.earscirev.2013.04.005.
- ^ a b Macharé, José; Devries, Thomas; Barron, John; Fourtanier, Élisabeth (1988). "Oligo-Miocene transgression along the Pacifie margin of South America: new paleontological and geological evidence from the Pisco basin (Peru)" (PDF). Geódynamique. 3 (1–2): 25–37.
- ^ a b Encinas, Alfonso; Pérez, Felipe; Nielsen, Sven; Finger, Kenneth L.; Valencia, Victor; Duhart, Paul (2014). "Geochronologic and paleontologic evidence for a Pacific–Atlantic connection during the late Oligocene–early Miocene in the Patagonian Andes (43–44°S)". Journal of South American Earth Sciences. 55: 1–18. Bibcode:2014JSAES..55....1E. doi:10.1016/j.jsames.2014.06.008. hdl:10533/130517.
- ^ Nielsen, S.N. (2005). "Cenozoic Strombidae, Aporrhaidae, and Struthiolariidae (Gastropoda, Stromboidea) from Chile: their significance to biogeography of faunas and climate of the south-east Pacific". Journal of Paleontology. 79: 1120–1130. doi:10.1666/0022-3360(2005)079[1120:csaasg]2.0.co;2. S2CID 130207579.
- ^ a b Guillame, Benjamin; Martinod, Joseph; Husson, Laurent; Roddaz, Martin; Riquelme, Rodrigo (2009). "Neogene uplift of central eastern Patagonia: Dynamic response to active spreading ridge subduction?". Tectonics. 28.
- ^ Cande, S.C.; Leslie, R.B. (1986). "Late Cenozoic Tectonics of the Southern Chile Trench". Journal of Geophysical Research: Solid Earth. 91 (B1): 471–496. Bibcode:1986JGR....91..471C. doi:10.1029/jb091ib01p00471.
- ^ Guillaume, Benjamin; Gautheron, Cécile; Simon-Labric, Thibaud; Martinod, Joseph; Roddaz, Martin; Douville, Eric (2013). "Dynamic topography control on Patagonian relief evolution as inferred from low temperature thermochronology". Earth and Planetary Science Letters. 3: 157–167. Bibcode:2013E&PSL.364..157G. doi:10.1016/j.epsl.2012.12.036.
- ^ Folguera, Andrés; Encinas, Alfonso; Echaurren, Andrés; Gianni, Guido; Orts, Darío; Valencia, Víctor; Carrasco, Gabriel (2018). "Constraints on the Neogene growth of the central Patagonian Andes at thelatitude of the Chile triple junction (45–47°S) using U/Pb geochronology insynorogenic strata". Tectonophysics. 744: 134–154. Bibcode:2018Tectp.744..134F. doi:10.1016/j.tecto.2018.06.011. hdl:11336/88399. S2CID 135214581.
- ^ Herold, L.; Huber, M.; Greenwood, D. R.; Müller, R. D.; Seton, M. (1 January 2011). "Early to Middle Miocene monsoon climate in Australia". Geology. 39 (1): 3–6. Bibcode:2011Geo....39....3H. doi:10.1130/G31208.1. Retrieved 14 July 2023.
- ^ Travouillon, K. J.; Archer, M.; Hand, S. J. (1 June 2012). "Early to middle Miocene monsoon climate in Australia: COMMENT". Geology. 40 (6): e273. Bibcode:2012Geo....40E.273T. doi:10.1130/G32600C.1.
- ^ a b c Groeneveld, Jeroen; Henderiks, Jorijntje; Renema, Willem; McHugh, Cecilia M.; De Vleeschouwer, David; Christensen, Beth A.; Fulthorpe, Craig S.; Reuning, Lars; Gallager, Stephen J.; Bogus, Kara; Auer, Gerald; Ishiwa, Takeshige; Expedition 356 Scientists (10 May 2017). "Australian shelf sediments reveal shifts in Miocene Southern Hemisphere westerlies". Science Advances. 3 (5): e1602567. Bibcode:2017SciA....3E2567G. doi:10.1126/sciadv.1602567. PMC 5425240. PMID 28508066.
{{cite journal}}
: CS1 maint: numeric names: authors list (link) - ^ Methner, Katharina; Campani, Marion; Fiebig, Jens; Löffler, Niklas; Kempf, Oliver; Mulch, Andreas (14 May 2020). "Middle Miocene long-term continental temperature change in and out of pace with marine climate records". Scientific Reports. 10 (1): 7989. Bibcode:2020NatSR..10.7989M. doi:10.1038/s41598-020-64743-5. PMC 7224295. PMID 32409728.
- ^ You, Y. (17 February 2010). "Climate-model evaluation of the contribution of sea-surface temperature and carbon dioxide to the Middle Miocene Climate Optimum as a possible analogue of future climate change". Australian Journal of Earth Sciences. 57 (2): 207–219. Bibcode:2010AuJES..57..207Y. doi:10.1080/08120090903521671. ISSN 0812-0099. S2CID 129238665. Retrieved 4 September 2023.
- ^ Retallack, Gregory J.; Conde, Giselle D. (June 2020). "Deep time perspective on rising atmospheric CO2". Global and Planetary Change. 189: 103177. Bibcode:2020GPC...18903177R. doi:10.1016/j.gloplacha.2020.103177. S2CID 216307251. Retrieved 5 June 2023.
- ^ a b c d e Scotese, Christopher R.; Song, Haijun; Mills, Benjamin J.W.; van der Meer, Douwe G. (April 2021). "Phanerozoic paleotemperatures: The earth's changing climate during the last 540 million years" (PDF). Earth-Science Reviews. 215: 103503. Bibcode:2021ESRv..21503503S. doi:10.1016/j.earscirev.2021.103503. ISSN 0012-8252. S2CID 233579194. Alt URL
- ^ Greenop, Rosanna; Sodian, Sindia M.; Henehan, Michael J.; Wilson, Paul A.; Lear, Caroline H.; Foster, Gavin L. (18 January 2019). "Orbital Forcing, Ice Volume, and CO2 Across the Oligocene-Miocene Transition". Paleoclimatology and Paleoceanography. 34 (3): 316–328. Bibcode:2019PaPa...34..316G. doi:10.1029/2018PA003420. S2CID 133785754. Retrieved 5 April 2023.
- ^ Roth-Nebelsick, A.; Utescher, T.; Mosbrugger, V.; Diester-Haass, L.; Walther, T. (20 March 2004). "Changes in atmospheric CO2 concentrations and climate from the Late Eocene to Early Miocene: palaeobotanical reconstruction based on fossil floras from Saxony, Germany". Palaeogeography, Palaeoclimatology, Palaeoecology. 205 (1–2): 43–67. Bibcode:2004PPP...205...43R. doi:10.1016/j.palaeo.2003.11.014. Retrieved 20 July 2023.
- ^ Goedert, Jean; Amiot, Romain; Arnaut-Godet, Florent; Cuny, Gilles; Fourel, François; Hernandez, Jean-Alexis; Pedreira-Segade, Ulysse; Lécuyer, Christophe (1 September 2017). "Miocene (Burdigalian) seawater and air temperatures estimated from the geochemistry of fossil remains from the Aquitaine Basin, France". Palaeogeography, Palaeoclimatology, Palaeoecology. 481: 14–28. Bibcode:2017PPP...481...14G. doi:10.1016/j.palaeo.2017.04.024. Retrieved 30 November 2022.
- ^ Zhang, Ran; Zhang, Zhongshi; Jiang, Dabang (23 October 2018). "Global Cooling Contributed to the Establishment of a Modern-Like East Asian Monsoon Climate by the Early Miocene". Geophysical Research Letters. 45 (21): 11, 941–11, 948. Bibcode:2018GeoRL..4511941Z. doi:10.1029/2018GL079930. S2CID 135353513. Retrieved 4 September 2023.
- ^ Zhang, Chunxia; Xiao, Guoqiao; Guo, Zhengtang; Wu, Haibin; Hao, Qingzhen (1 May 2015). "Evidence of late early Miocene aridification intensification in the Xining Basin caused by the northeastern Tibetan Plateau uplift". Global and Planetary Change. 128: 31–46. Bibcode:2015GPC...128...31Z. doi:10.1016/j.gloplacha.2015.02.002. ISSN 0921-8181. Retrieved 12 January 2024 – via Elsevier Science Direct.
- ^ Greenop, Rosanna; Foster, Gavin L.; Wilson, Paul A.; Lear, Caroline H. (11 August 2014). "Middle Miocene climate instability associated with high-amplitude CO2 variability". Paleoceanography and Paleoclimatology. 29 (9): 845–853. Bibcode:2014PalOc..29..845G. doi:10.1002/2014PA002653. S2CID 129813700. Retrieved 5 April 2023.
- ^ You, Y.; Huber, M.; Müller, R. D.; Poulsen, C. J.; Ribbe, J. (19 February 2009). "Simulation of the Middle Miocene Climate Optimum". Geophysical Research Letters. 36 (4): 1–5. Bibcode:2009GeoRL..36.4702Y. doi:10.1029/2008GL036571. ISSN 0094-8276. S2CID 17326204. Retrieved 4 September 2023.
- ^ Armstrong McKay, David I.; Tyrrell, Toby; Wilson, Paul A.; Foster, Gavin L. (1 October 2014). "Estimating the impact of the cryptic degassing of Large Igneous Provinces: A mid-Miocene case-study". Earth and Planetary Science Letters. 403: 254–262. Bibcode:2014E&PSL.403..254A. doi:10.1016/j.epsl.2014.06.040. Retrieved 29 April 2023.
- ^ Holbourn, Ann; Kuhnt, Wolfgang; Kochhann, Karlos G.D.; Andersen, Nils; Sebastian Meier, K.J. (1 February 2015). "Global perturbation of the carbon cycle at the onset of the Miocene Climatic Optimum". Geology. 43 (2): 123–126. Bibcode:2015Geo....43..123H. doi:10.1130/G36317.1. ISSN 1943-2682. Retrieved 4 September 2023.
- ^ Goto, Kosuke T.; Tejada, Maria Luisa G.; Tajika, Eiichi; Suzuki, Katsuhiko (26 January 2023). "Enhanced magmatism played a dominant role in triggering the Miocene Climatic Optimum". Communications Earth & Environment. 4 (1): 21. Bibcode:2023ComEE...4...21G. doi:10.1038/s43247-023-00684-x. ISSN 2662-4435. Retrieved 26 November 2023.
- ^ Henrot, A.-J.; François, L.; Favre, E.; Butzin, M.; Ouberdous, M.; Munhoven, G. (21 October 2010). "Effects of CO2, continental distribution, topography and vegetation changes on the climate at the Middle Miocene: a model study". Climate of the Past. 6 (5): 675–694. Bibcode:2010CliPa...6..675H. doi:10.5194/cp-6-675-2010. Retrieved 21 April 2023.
- ^ Goldner, A.; Herold, N.; Huber, M. (13 March 2014). "The challenge of simulating the warmth of the mid-Miocene climatic optimum in CESM1". Climate of the Past. 10 (2): 523–536. Bibcode:2014CliPa..10..523G. doi:10.5194/cp-10-523-2014. ISSN 1814-9332. Retrieved 4 September 2023.
- ^ Kroh, Andreas (14 September 2007). "Climate changes in the Early to Middle Miocene of the Central Paratethys and the origin of its echinoderm fauna". Palaeogeography, Palaeoclimatology, Palaeoecology. Miocene Climate in Europe - patterns and evolution. First synthesis of NECLIME. 253 (1): 169–207. Bibcode:2007PPP...253..169K. doi:10.1016/j.palaeo.2007.03.039. ISSN 0031-0182. Retrieved 12 October 2023.
- ^ Liu, Chang; Clift, Peter D.; Giosan, Liviu; Miao, Yunfa; Warny, Sophie; Wan, Shiming (1 July 2019). "Paleoclimatic evolution of the SW and NE South China Sea and its relationship with spectral reflectance data over various age scales". Palaeogeography, Palaeoclimatology, Palaeoecology. 525: 25–43. Bibcode:2019PPP...525...25L. doi:10.1016/j.palaeo.2019.02.019. S2CID 135413974. Retrieved 14 November 2022.
- ^ Warny, Sophie; Askin, Rosemary A.; Hannah, Michael J.; Mohr, Barbara A.R.; Raine, J. Ian; Harwood, David M.; Florindo, Fabio; the SMS Science Team (1 October 2009). "Palynomorphs from a sediment core reveal a sudden remarkably warm Antarctica during the middle Miocene". Geology. 37 (10): 955–958. Bibcode:2009Geo....37..955W. doi:10.1130/G30139A.1. ISSN 1943-2682. Retrieved 4 September 2023.
- ^ Kochhann, Karlos G. D.; Holbourn, Ann; Kuhnt, Wolfgang; Channell, James E. T.; Lyle, Mitch; Shackford, Julia K.; Wilkens, Roy H.; Andersen, Nils (22 August 2016). "Eccentricity pacing of eastern equatorial Pacific carbonate dissolution cycles during the Miocene Climatic Optimum: ECCENTRICITY-PACED DISSOLUTION CYCLES". Paleoceanography and Paleoclimatology. 31 (9): 1176–1192. doi:10.1002/2016PA002988. Retrieved 4 September 2023.
- ^ Shevenell, Amelia E.; Kennett, James P.; Lea, David W. (17 September 2004). "Middle Miocene Southern Ocean Cooling and Antarctic Cryosphere Expansion". Science. 305 (5691): 1766–1770. Bibcode:2004Sci...305.1766S. doi:10.1126/science.1100061. ISSN 0036-8075. PMID 15375266. S2CID 27369039. Retrieved 5 April 2023.
- ^ Holbourn, A.; Kuhnt, W.; Lyle, M.; Schneider, L.; Romero, O.; Andersen, N. (1 January 2014). "Middle Miocene climate cooling linked to intensification of eastern equatorial Pacific upwelling". Geology. 42 (1): 19–22. Bibcode:2014Geo....42...19H. doi:10.1130/G34890.1. ISSN 0091-7613. Retrieved 4 September 2023.
- ^ Super, James R.; Thomas, Ellen; Pagani, Mark; Huber, Matthew; O'Brien, Charlotte; Hull, Pincelli M. (26 April 2018). "North Atlantic temperature and pCO2 coupling in the early-middle Miocene". Geology. 46 (6): 519–522. Bibcode:2018Geo....46..519S. doi:10.1130/G40228.1. ISSN 0091-7613. Retrieved 4 September 2023.
- ^ Woodruff, Fay; Savin, Samuel (December 1991). "Mid-Miocene isotope stratigraphy in the deep sea: High-resolution correlations, paleoclimatic cycles, and sediment preservation". Paleoceanography and Paleoclimatology. 6 (6): 755–806. Bibcode:1991PalOc...6..755W. doi:10.1029/91PA02561. Retrieved 4 September 2023.
- ^ Mathew, Manoj; Makhankova, Adelya; Menier, David; Sautter, Benjamin; Betzler, Christian; Pierson, Bernard (28 April 2020). "The emergence of Miocene reefs in South China Sea and its resilient adaptability under varying eustatic, climatic and oceanographic conditions". Scientific Reports. 10 (1): 7141. Bibcode:2020NatSR..10.7141M. doi:10.1038/s41598-020-64119-9. PMC 7189246. PMID 32346046. Retrieved 23 April 2023.
- ^ Flower, Benjamin P.; Kennett, James P. (April 1994). "The middle Miocene climatic transition: East Antarctic ice sheet development, deep ocean circulation and global carbon cycling". Palaeogeography, Palaeoclimatology, Palaeoecology. 108 (3–4): 537–555. Bibcode:1994PPP...108..537F. doi:10.1016/0031-0182(94)90251-8. Retrieved 4 September 2023.
- ^ Tian, Jun; Zhao, Quanhong; Wang, Pinxian; Li, Qianyu; Cheng, Xinrong (September 2008). "Astronomically modulated Neogene sediment records from the South China Sea: NEOGENE BENTHIC ISOTOPES". Paleoceanography and Paleoclimatology. 23 (3): 1–20. doi:10.1029/2007PA001552. Retrieved 19 September 2023.
- ^ Holbourn, Ann; Kuhnt, Wolfgang; Clemens, Steven; Prell, Warren; Andersen, Nils (11 November 2013). "Middle to late Miocene stepwise climate cooling: Evidence from a high-resolution deep water isotope curve spanning 8 million years: MIOCENE BENTHIC ISOTOPES". Paleoceanography and Paleoclimatology. 28 (4): 688–699. doi:10.1002/2013PA002538. S2CID 128368245. Retrieved 4 September 2023.
- ^ Zhang, Rui; Li, Xiaojuan; Xu, Yong; Li, Jianxian; Sun, Lu; Yue, Leping; Pan, Feng; Xian, Feng; Wei, Xiaohao; Cao, Yuge (10 January 2022). "The 173-kyr Obliquity Cycle Pacing the Asian Monsoon in the Eastern Chinese Loess Plateau From Late Miocene to Pliocene". Geophysical Research Letters. 49 (2). Bibcode:2022GeoRL..4997008Z. doi:10.1029/2021GL097008. S2CID 245868256. Retrieved 20 March 2023.
- ^ Retallack, Gregory J. (4 November 2004). "Late Miocene climate and life on land in Oregon within a context of Neogene global change". Palaeogeography, Palaeoclimatology, Palaeoecology. 214 (1): 97–123. doi:10.1016/j.palaeo.2004.07.024. ISSN 0031-0182. Retrieved 12 January 2024 – via Elsevier Science Direct.
- ^ Mao, Xuegang; Retallack, Gregory (15 January 2019). "Late Miocene drying of central Australia". Palaeogeography, Palaeoclimatology, Palaeoecology. 514: 292–304. Bibcode:2019PPP...514..292M. doi:10.1016/j.palaeo.2018.10.008. S2CID 135124769. Retrieved 14 July 2023.
- ^ Lee, Jongmin; Kim, Sunghan; Lee, Jae Il; Cho, Hyen Goo; Phillips, Stephen C.; Khim, Bo-Kyeun (15 December 2020). "Monsoon-influenced variation of clay mineral compositions and detrital Nd-Sr isotopes in the western Andaman Sea (IODP Site U1447) since the late Miocene". Palaeogeography, Palaeoclimatology, Palaeoecology. 538: 109339. Bibcode:2020PPP...53809339L. doi:10.1016/j.palaeo.2019.109339. S2CID 202179283. Retrieved 7 July 2023.
- ^ Matsuzaki, Kenji M.; Ikeda, Masayuki; Tada, Ryuji (20 July 2022). "Weakened pacific overturning circulation, winter monsoon dominance and tectonism re-organized Japan Sea paleoceanography during the Late Miocene global cooling". Scientific Reports. 12 (1): 11396. Bibcode:2022NatSR..1211396M. doi:10.1038/s41598-022-15441-x. PMC 9300741. PMID 35859095.
- ^ Larsen, H. C.; Saunders, A. D.; Clift, P. D.; Beget, J.; Wei, W.; Spezzaferri, S. (13 May 1994). "Seven Million Years of Glaciation in Greenland". Science. 264 (5161): 952–955. Bibcode:1994Sci...264..952L. doi:10.1126/science.264.5161.952. PMID 17830083. S2CID 10031704.
- ^ John, Kristen E. K. St.; Krissek, Lawrence A. (28 June 2008). "The late Miocene to Pleistocene ice-rafting history of southeast Greenland". Boreas. 31 (1): 28–35. doi:10.1111/j.1502-3885.2002.tb01053.x. S2CID 128606939.
- ^ Funder, Svend; Abrahamsen, Niels; Bennike, Ole; Feyling-Hanssen, Rolf W. (1 August 1985). "Forested Arctic: Evidence from North Greenland". Geology. 13 (8): 542–546. Bibcode:1985Geo....13..542F. doi:10.1130/0091-7613(1985)13<542:FAEFNG>2.0.CO;2.
- ^ Wang, Xue-Lian; Wang, Zi-Xi; Li, Rui-Yun; Deng, Peng; Ma, Li; Sun, Bai-Nian (January 2016). "Vein density of angiosperms as a paleoclimate proxy: a case study using fossil leaves of Zelkova and Machilus". Palaeoworld. 25 (1): 60–66. doi:10.1016/j.palwor.2015.11.002. Retrieved 20 July 2024 – via Elsevier Science Direct.
- ^ Jacobs, Bonnie Fine (8 April 2016). "Estimation of low-latitude paleoclimates using fossil angiosperm leaves: examples from the Miocene Tugen Hills, Kenya". Paleobiology. 28 (3): 399–421. Bibcode:2002Pbio...28..399J. doi:10.1666/0094-8373(2002)028<0399:EOLLPU>2.0.CO;2. JSTOR 3595489. S2CID 198156844. Retrieved 16 June 2023.
- ^ Brown, Rachel M.; Chalk, Thomas B.; Crocker, Anya J.; Wilson, Paul A.; Foster, Gavin L. (25 July 2022). "Late Miocene cooling coupled to carbon dioxide with Pleistocene-like climate sensitivity". Nature Geoscience. 15 (8): 664–670. Bibcode:2022NatGe..15..664B. doi:10.1038/s41561-022-00982-7. hdl:10037/29226. S2CID 251043167. Retrieved 8 December 2022.
- ^ Tanner, Thomas; Hernández-Almeida, Iván; Drury, Anna Joy; Guitián, José; Stoll, Heather (10 December 2020). "Decreasing Atmospheric CO2 During the Late Miocene Cooling". Paleoceanography and Paleoclimatology. 35 (12). Bibcode:2020PaPa...35.3925T. doi:10.1029/2020PA003925. S2CID 230534117. Retrieved 17 March 2023.
- ^ Wen, Yixiong; Zhang, Laiming; Holbourn, Ann E.; Zhu, Chenguang; Huntington, Katharine W.; Jin, Tianjie; Li, Yalin; Wang, Chengshan (23 January 2013). "CO2-forced Late Miocene cooling and ecosystem reorganizations in East Asia". Proceedings of the National Academy of Sciences of the United States of America. 120 (5): e2214655120. doi:10.1073/pnas.2214655120. PMC 9945954. PMID 36689658.
- ^ a b Qin, Jie; Zhang, Rui; Kravchinsky, Vadim A.; Valet, Jean-Pierre; Sagnotti, Leonardo; Li, Jianxing; Xu, Yong; Anwar, Taslima; Yue, Leping (2 April 2022). "1.2 Myr Band of Earth-Mars Obliquity Modulation on the Evolution of Cold Late Miocene to Warm Early Pliocene Climate". Solid Earth. 127 (4). Bibcode:2022JGRB..12724131Q. doi:10.1029/2022JB024131. S2CID 247933545. Retrieved 24 November 2022.
- ^ Herbert, Timothy D.; Lawrence, Kira T.; Tzanova, Alexandrina; Peterson, Laura Cleaveland; Caballero-Gill, Rocio; Kelly, Christopher S. (26 September 2016). "Late Miocene global cooling and the rise of modern ecosystems". Nature Geoscience. 9 (11): 843–847. Bibcode:2016NatGe...9..843H. doi:10.1038/ngeo2813. Retrieved 17 March 2023.
- ^ Mejía, Luz María; Méndez-Vicente, Ana; Abrevaya, Lorena; Lawrence, Kira T.; Ladlow, Caroline; Bolton, Clara; Cacho, Isabel; Stoll, Heather (1 December 2017). "A diatom record of CO2 decline since the late Miocene". Earth and Planetary Science Letters. 479: 18–33. Bibcode:2017E&PSL.479...18M. doi:10.1016/j.epsl.2017.08.034.
- ^ Drury, Anna Joy; Westerhold, Thomas; Frederichs, Thomas; Tian, Jun; Wilkens, Roy; Channell, James E.T.; Evans, Helen; John, Cédric M.; Lyle, Mitch; Röhl, Ursula (1 October 2017). "Late Miocene climate and time scale reconciliation: Accurate orbital calibration from a deep-sea perspective". Earth and Planetary Science Letters. 475: 254–266. doi:10.1016/j.epsl.2017.07.038. Retrieved 20 July 2024 – via Elsevier Science Direct.
- ^ Hodell, David A.; Curtis, Jason H.; Sierro, Francisco J.; Raymo, Maureen E. (April 2004). "Correlation of Late Miocene to Early Pliocene sequences between the Mediterranean and North Atlantic". Paleoceanography and Paleoclimatology. 16 (2): 164–178. doi:10.1029/1999PA000487. ISSN 0883-8305. Retrieved 19 September 2023.
- ^ De Vleeschouwer, David; Drury, Anna Joy; Vahlenkamp, Maximilian; Rochholz, Fiona; Liebrand, Diederik; Pälike, Heiko (6 October 2020). "High-latitude biomes and rock weathering mediate climate–carbon cycle feedbacks on eccentricity timescales". Nature Communications. 11 (1): 5013. Bibcode:2020NatCo..11.5013D. doi:10.1038/s41467-020-18733-w. PMC 7538577. PMID 33024102.
- ^ Ao, Hong; Rohling, Eelco J.; Zhang, Ran; Roberts, Andrew P.; Holbourn, Ann E.; Ladant, Jean-Baptiste; Dupont-Nivet, Guillaume; Kuhnt, Wolfgang; Zhang, Peng; Wu, Feng; Dekkers, Mark J.; Liu, Qingsong; Liu, Zhonghui; Xu, Yong; Poulsen, Christopher J. (26 November 2021). "Global warming-induced Asian hydrological climate transition across the Miocene–Pliocene boundary". Nature Communications. 12 (1): 6935. Bibcode:2021NatCo..12.6935A. doi:10.1038/s41467-021-27054-5. ISSN 2041-1723. PMC 8626456. PMID 34836960.
- ^ Han, Wenxia; Fang, Xiaomin; Berger, André; Yin, Qiuzhen (22 December 2011). "An astronomically tuned 8.1 Ma eolian record from the Chinese Loess Plateau and its implication on the evolution of Asian monsoon". Journal of Geophysical Research. 116 (D24): 1–13. Bibcode:2011JGRD..11624114H. doi:10.1029/2011JD016237. Retrieved 20 March 2023.
- ^ Carolin, Nora; Bajpai, Sunil; Maurya, Abhayanand Singh; Schwarzhans, Werner (2022). "New perspectives on late Tethyan Neogene biodiversity development of fishes based on Miocene (~ 17 Ma) otoliths from southwestern India". PalZ. 97: 43–80. doi:10.1007/s12542-022-00623-9. S2CID 249184395.
- ^ Fenton, Isabel S.; Aze, Tracy; Farnsworth, Alexander; Valdes, Paul; Saupe, Erin E. (15 February 2023). "Origination of the modern-style diversity gradient 15 million years ago". Nature. 614 (7949): 708–712. Bibcode:2023Natur.614..708F. doi:10.1038/s41586-023-05712-6. PMID 36792825. S2CID 256899993. Archived from the original on 12 April 2023. Retrieved 12 April 2023.
{{cite journal}}
: CS1 maint: bot: original URL status unknown (link) - ^ Attorre, F.; Francesconi, F.; Taleb, N.; Scholte, P.; Saed, A.; Alfo, M.; Bruno, F. (2007). "Will dragonblood survive the next period of climate change? Current and future potential distribution of Dracaena cinnabari (Socotra, Yemen)". Biological Conservation. 138 (3–4): 430–439. Bibcode:2007BCons.138..430A. doi:10.1016/j.biocon.2007.05.009. hdl:11573/234206.
- ^ Retallack, Gregory (2001). "Cenozoic Expansion of Grasslands and Climatic Cooling" (PDF). The Journal of Geology. 109 (4). University of Chicago Press: 407–426. Bibcode:2001JG....109..407R. doi:10.1086/320791. S2CID 15560105. Archived from the original (PDF) on 2013-05-06.
- ^ Osborne, C.P.; Beerling, D.J. (2006). "Nature's green revolution: the remarkable evolutionary rise of C4 plants". Philosophical Transactions of the Royal Society B: Biological Sciences. 361 (1465): 173–194. doi:10.1098/rstb.2005.1737. PMC 1626541. PMID 16553316.
- ^ Wolfram M. Kürschner, Zlatko Kvacek & David L. Dilcher (2008). "The impact of Miocene atmospheric carbon dioxide fluctuations on climate and the evolution of terrestrial ecosystems". Proceedings of the National Academy of Sciences of the United States of America. 105 (2): 449–53. Bibcode:2008PNAS..105..449K. doi:10.1073/pnas.0708588105. PMC 2206556. PMID 18174330.
- ^ Keeley, Jon E.; Rundel, Philip W. (28 April 2005). "Fire and the Miocene expansion of C4 grasslands". Ecology Letters. 8 (7): 683–690. Bibcode:2005EcolL...8..683K. doi:10.1111/j.1461-0248.2005.00767.x. Retrieved 21 March 2023.
- ^ Du, Jinlong; Tian, Jun; Ma, Wentao (15 April 2022). "The Late Miocene Carbon Isotope Shift driven by synergetic terrestrial processes: A box-model study". Earth and Planetary Science Letters. 584: 117457. Bibcode:2022E&PSL.58417457D. doi:10.1016/j.epsl.2022.117457. ISSN 0012-821X. S2CID 247307062. Retrieved 30 December 2023 – via Elsevier Science Direct.
- ^ Susanne S. Renner (2011). "Living fossil younger than thought". Science. 334 (6057): 766–767. Bibcode:2011Sci...334..766R. doi:10.1126/science.1214649. PMID 22076366. S2CID 206537832.
- ^ "Eucalyptus fossils in New Zealand - the thin end of the wedge - Mike Pole". 2014-09-22.
- ^ Steven M. Stanley (1999). Earth System History. New York: Freeman. pp. 525–526. ISBN 0-7167-2882-6.
- ^ Furió, Marc; Casanovas-Vilar, Isaac; van den Hoek Ostende, Lars W. (1 May 2011). "Predictable structure of Miocene insectivore (Lipotyphla) faunas in Western Europe along a latitudinal gradient". Palaeogeography, Palaeoclimatology, Palaeoecology. The Neogene of Eurasia: Spatial gradients and temporal trends - The second synthesis of NECLIME. 304 (3): 219–229. Bibcode:2011PPP...304..219F. doi:10.1016/j.palaeo.2010.01.039. ISSN 0031-0182. Retrieved 12 January 2024 – via Elsevier Science Direct.
- ^ Matsumoto R, Evans SE (2010). "Choristoderes and the freshwater assemblages of Laurasia". Journal of Iberian Geology. 36 (2): 253–274. Bibcode:2010JIbG...36..253M. doi:10.5209/rev_jige.2010.v36.n2.11.
- ^ Rougier, Guillermo W.; Wible, John R.; Beck, Robin M. D.; Apesteguía, Sebastian (2012-12-04). "The Miocene mammal Necrolestes demonstrates the survival of a Mesozoic nontherian lineage into the late Cenozoic of South America". Proceedings of the National Academy of Sciences of the United States of America. 109 (49): 20053–20058. Bibcode:2012PNAS..10920053R. doi:10.1073/pnas.1212997109. ISSN 0027-8424. PMC 3523863. PMID 23169652.
- ^ Nicolás R. Chimento, Federico L. Agnolin and Fernando E. Novas (2012). "The Patagonian fossil mammal Necrolestes: a Neogene survivor of Dryolestoidea" (PDF). Revista del Museo Argentino de Ciencias Naturales. Nueva Serie. 14 (2): 261–306. Archived from the original (PDF) on 2013-11-04. Retrieved 2017-08-08.
- ^ Furió, Marc; Ruiz-Sánchez, Francisco J.; Crespo, Vicente D.; Freudenthal, Matthijs; Montoya, Plinio (July 2012). "The southernmost Miocene occurrence of the last European herpetotheriid Amphiperatherium frequens (Metatheria, Mammalia)". Comptes Rendus Palevol. 11 (5): 371–377. Bibcode:2012CRPal..11..371F. doi:10.1016/j.crpv.2012.01.004.
- ^ Bennett, C. Verity; Upchurch, Paul; Goin, Francisco J.; Goswami, Anjali (2018-02-06). "Deep time diversity of metatherian mammals: implications for evolutionary history and fossil-record quality". Paleobiology. 44 (2): 171–198. Bibcode:2018Pbio...44..171B. doi:10.1017/pab.2017.34. ISSN 0094-8373. S2CID 46796692.
- ^ Crespo, Vicente D.; Goin, Francisco J.; Pickford, Martin (2022-06-03). "The last African metatherian". Fossil Record. 25 (1): 173–186. doi:10.3897/fr.25.80706. ISSN 2193-0074. S2CID 249349445.
- ^ Yirka, Bob (August 15, 2012). "New genetic data shows humans and great apes diverged earlier than thought". phys.org.
- ^ Begun, David. "Fossil Record of Miocene Hominoids" (PDF). University of Toronto. Archived from the original (PDF) on October 30, 2013. Retrieved July 11, 2014.
- ^ van der Made, Jan (1 April 2014). "Late Pleistocene European and Late Miocene African accelerations of faunal change in relation to the climate and as a background to human evolution". Quaternary International. 326–327: 431–447. doi:10.1016/j.quaint.2013.12.003. Retrieved 20 July 2024 – via Elsevier Science Direct.
- ^ Merceron, Gildas; Kaiser, Thomas M.; Kostopoulos, Dimitris S.; Schulz, Ellen (2 June 2010). "Ruminant diets and the Miocene extinction of European great apes". Proceedings of the Royal Society B: Biological Sciences. 277 (1697): 3105–3112. doi:10.1098/rspb.2010.0523. ISSN 0962-8452. PMC 2982054. PMID 20519220. Retrieved 20 July 2024.
- ^ a b Holman, J. Alan (2000). Fossil Snakes of North America (First ed.). Bloomington, IN: Indiana University Press. pp. 284–323. ISBN 0-253-33721-6.
- ^ Li, Qijia; Deng, Weiyudong; Wappler, Torsten; Utescher, Torsten; Maslova, Natalia; Liu, Yusheng (Christopher); Jia, Hui; Song, Chengyu; Su, Tao; Quan, Cheng (February 2022). "High frequency of arthropod herbivore damage in the Miocene Huaitoutala flora from the Qaidam Basin, northern Tibetan Plateau". Review of Palaeobotany and Palynology. 297: 104569. doi:10.1016/j.revpalbo.2021.104569. Retrieved 20 July 2024 – via Elsevier Science Direct.
- ^ Wang, Menglin; Hellemans, Simon; Buček, Aleš; Kanao, Taisuke; Arora, Jigyasa; Clitheroe, Crystal; Rafanomezantsoa, Jean‐Jacques; Fisher, Brian L.; Scheffrahn, Rudolf; Sillam‐Dussès, David; Roisin, Yves; Šobotník, Jan; Bourguignon, Thomas (21 April 2023). "Neoisoptera repeatedly colonised Madagascar after the Middle Miocene climatic optimum". Ecography. 2023 (7). doi:10.1111/ecog.06463. ISSN 0906-7590. Retrieved 4 June 2024.
- ^ Petrick, Benjamin; Reuning, Lars; Auer, Gerald; Zhang, Yige; Pfeiffer, Miriam; Schwark, Lorenz (10 March 2023). "Warm, not cold temperatures contributed to a Late Miocene reef decline in the Coral Sea". Scientific Reports. 13 (1): 4015. Bibcode:2023NatSR..13.4015P. doi:10.1038/s41598-023-31034-8. ISSN 2045-2322. PMC 10006184. PMID 36899047.
- ^ Peter Klimley & David Ainley (1996). Great White Sharks: the Biology of Carcharodon carcharias. Academic Press. ISBN 0-12-415031-4. Archived from the original on 2012-10-12. Retrieved 2011-08-12.
- ^ Dooley, Alton C.; Fraser, Nicholas C.; Luo, Zhe-Xi (2004). "The earliest known member of the rorqual—gray whale clade (Mammalia, Cetacea)". Journal of Vertebrate Paleontology. 24 (2): 453–463. Bibcode:2004JVPal..24..453D. doi:10.1671/2401. ISSN 0272-4634. S2CID 84970052.
- ^ a b Olivier Lambert; Giovanni Bianucci; Klaas Post; Christian de Muizon; Rodolfo Salas-Gismondi; Mario Urbina; Jelle Reumer (2010). "The giant bite of a new raptorial sperm whale from the Miocene epoch of Peru". Nature. 466 (7302): 105–108. Bibcode:2010Natur.466..105L. doi:10.1038/nature09067. PMID 20596020. S2CID 4369352.
- ^ Orangel A. Aguilera, Douglas Riff & Jean Bocquentin-Villanueva (2006). "A new giant Pusussaurus (Crocodyliformes, Alligatoridae) from the Upper Miocene Urumaco Formation, Venezuela" (PDF). Journal of Systematic Palaeontology. 4 (3): 221–232. Bibcode:2006JSPal...4..221A. doi:10.1017/S147720190600188X. S2CID 85950121. Archived from the original (PDF) on 2012-03-29.
- ^ a b Cidade, Giovanne M.; Fortier, Daniel; Hsiou, Annie Schmaltz (March 2019). "The crocodylomorph fauna of the Cenozoic of South America and its evolutionary history: a review". Journal of South American Earth Sciences. 90: 392–411. Bibcode:2019JSAES..90..392C. doi:10.1016/j.jsames.2018.12.026. S2CID 134902094.
- ^ Wilberg, Eric W.; Turner, Alan H.; Brochu, Christopher A. (2019-01-24). "Evolutionary structure and timing of major habitat shifts in Crocodylomorpha". Scientific Reports. 9 (1): 514. Bibcode:2019NatSR...9..514W. doi:10.1038/s41598-018-36795-1. ISSN 2045-2322. PMC 6346023. PMID 30679529.
- ^ Lawrence G. Barnes & Kiyoharu Hirota (1994). "Miocene pinnipeds of the otariid subfamily Allodesminae in the North Pacific Ocean: systematics and relationships". Island Arc. 3 (4): 329–360. Bibcode:1994IsArc...3..329B. doi:10.1111/j.1440-1738.1994.tb00119.x.
- ^ Drake, Henrik; Roberts, Nick M. W.; Reinhardt, Manuel; Whitehouse, Martin; Ivarsson, Magnus; Karlsson, Andreas; Kooijman, Ellen; Kielmann-Schmitt, Melanie (3 June 2021). "Biosignatures of ancient microbial life are present across the igneous crust of the Fennoscandian shield". Communications Earth & Environment. 2: 1–13. doi:10.1038/s43247-021-00170-2. S2CID 235307116. Retrieved 14 January 2023.
- ^ Lazarus, David; Barron, John; Renaudie, Johan; Diver, Patrick; Türke, Andreas (22 January 2014). "Cenozoic Planktonic Marine Diatom Diversity and Correlation to Climate Change". PLOS ONE. 9 (1): e84857. Bibcode:2014PLoSO...984857L. doi:10.1371/journal.pone.0084857. PMC 3898954. PMID 24465441.
- ^ a b Kenneth G. Miller & Richard G. Fairbanks (1983). "Evidence for Oligocene−Middle Miocene abyssal circulation changes in the western North Atlantic". Nature. 306 (5940): 250–253. Bibcode:1983Natur.306..250M. doi:10.1038/306250a0. S2CID 4337071.
- ^ Jiang, Shijun; Wise Jr., Sherwood W.; Wang, Yang (2007). Teagle, D.A.H.; Wilson, D.S.; Acton, G.D.; Vanko, D.A. (eds.). Proceedings of the Ocean Drilling Program, 206 Scientific Results. Vol. 206. Ocean Drilling Program. pp. 1–24. doi:10.2973/odp.proc.sr.206.013.2007.
- ^ Errázuriz-Henao, Carlos; Gómez-Tuena, Arturo; Parolari, Mattia; Weber, Marion (November 2022). "Climate-driven compositional modifications of arc volcanoes along the East Equatorial Pacific Margin — The magmatic response to a cooling planet". Earth-Science Reviews. 234: 104228. Bibcode:2022ESRv..23404228E. doi:10.1016/j.earscirev.2022.104228. Retrieved 26 November 2023.
- ^ Bouley S, Baratoux D, Baratoux L, Colas F, Dauvergne J, Losiak A, Vaubaillon J, Bourdeille C, Jullien A, Ibadinov K (2011). "Karakul: a young complex impact crater in the Pamir, Tajikistan". American Geophysical Union Fall Meeting Abstracts. 2011: P31A–1701. Bibcode:2011AGUFM.P31A1701B.
- ^ Gurov EP, Gurova HP, Rakitskaya RB, Yamnichenko, AYu (1993). The Karakul depression in Pamirs - the first impact structure in central Asia (PDF). Lunar and Planetary Inst., Twenty-Fourth Lunar and Planetary Science Conference. pp. 591–592. Bibcode:1993LPI....24..591G.
Further reading
[edit]- Cox, C. Barry & Moore, Peter D. (1993): Biogeography. An ecological and evolutionary approach (5th ed.). Blackwell Scientific Publications, Cambridge. ISBN 0-632-02967-6
- Ogg, Jim (2004): "Overview of Global Boundary Stratotype Sections and Points (GSSP's)". Retrieved 2006-04-30.
External links
[edit]
